The field of soft robotics seeks to create compliant, adaptive actuators that mimic the remarkable abilities of biological systems. Conventional robotic actuators, built from rigid components and powered by electric motors or hydraulics, excel at speed and precision but struggle to match the versatility and robustness of their natural counterparts. Soft actuators, in contrast, leverage the inherent compliance of flexible materials like polymers and elastomers to safely interact with delicate objects and adapt to unstructured environments. |
However, the physical mechanisms typically used to drive untethered soft actuators—such as swelling of hydrogels, thermal expansion, or liquid crystal realignment—suffer from slow response times on the order of seconds to minutes. |
Prior attempts to accelerate soft actuator performance using magnetic fields showed promise but faced daunting fabrication challenges. Embedding magnetic particles with precisely controlled orientations into soft polymer matrices requires specialized 3D printing equipment and strong external fields, complicating production. |
As an alternative, some researchers incorporated prefabricated rigid magnetic components directly into soft actuators. But without a general design approach to optimize the integration of hard and soft materials, these actuators could not fully exploit their hybrid architecture to enhance speed and strength while retaining flexibility and durability. |
Now, a team of engineers has taken inspiration from nature to devise a new paradigm for building high-performance soft magnetic actuators. As reported in a recent paper published in Advanced Functional Materials ("Ultrafast Biomimetic Untethered Soft Actuators with Bone-In-Flesh Constructs Actuated by Magnetic Field"), they demonstrate how strategically combining stiff magnetic "bones" with elastomeric "flesh" enables magnetically powered soft machines that actuate with unprecedented agility and precision while withstanding significant mechanical abuse. |
This bioinspired approach, which the authors dub "bone-in-flesh constructs," marks an important step toward soft actuators that begin to approach the capabilities of living systems. |
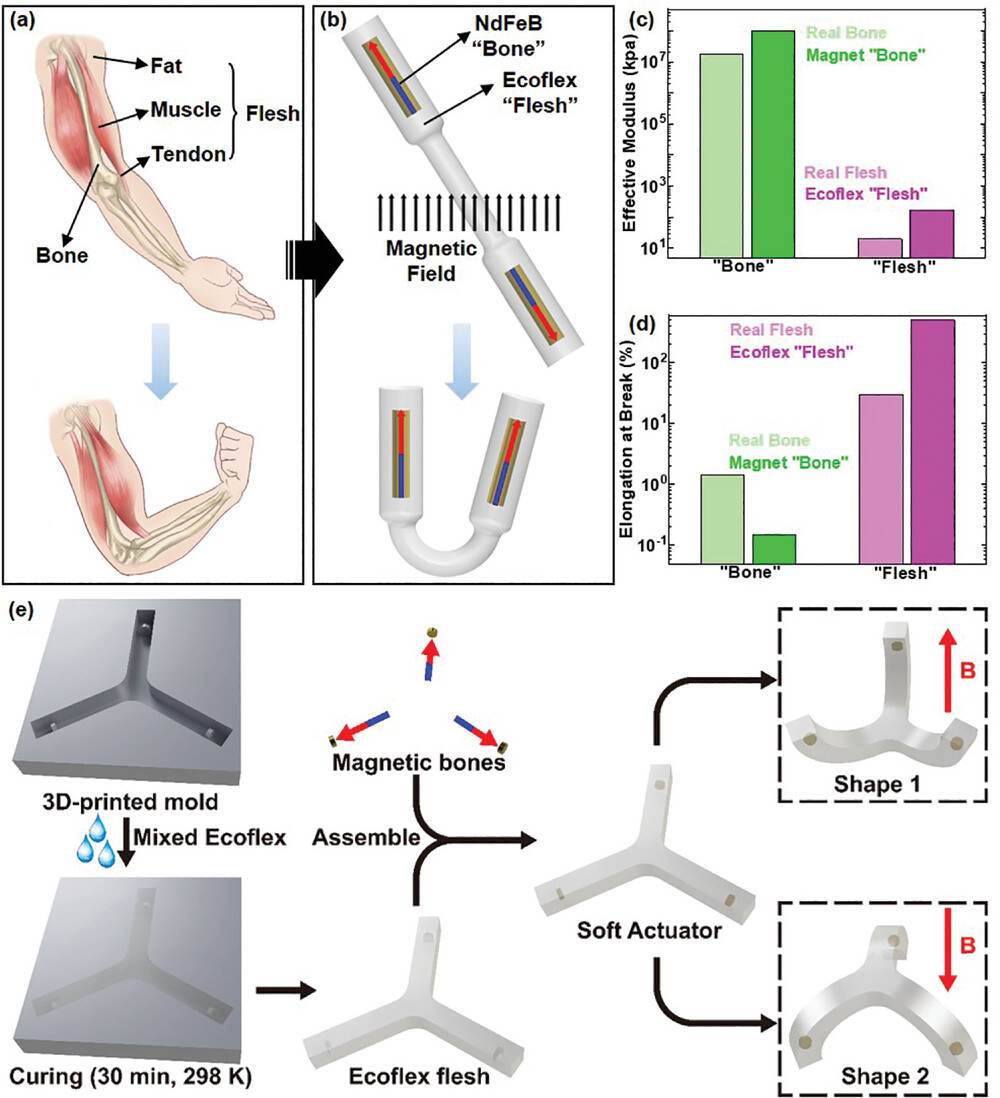 |
a) The flexing of a human arm, featuring the bones and flesh components. b) Schematics of the working mechanism of a bioinspired bone-inflesh actuator, with magnets as “bones” and elastomers as “flesh”. c) Effective modulus, and d) deformability (evaluated by the amount of elongation at break) of “bone” and “flesh” components in human body and in the proposed actuators. e) Fabrication procedures of bone-in-flesh soft actuators. Mixed Ecoflex is poured into the 3D printed ABS mold, and cured in room temperature for 30 min. The cured Ecoflex flesh is then taken out from the model and inserted with the NdFeB magnetic “bones”. After that, the cavities for insertion are enclosed with more Ecoflex. The fabricated soft actuator can deform into different shapes under different external magnetic fields. (Reprinted from DOI:10.1002/adfm.202401159, CC BY) |
The key insight behind bone-in-flesh design is that biological structures like human limbs integrate rigid skeletal elements and soft tissues in ways that synergistically enhance functionality. Bones enable fast, precise movements by acting as stiff levers, while compliant muscles, tendons, and skin protect the skeleton from impacts and provide a forgiving interface with the environment. |
The researchers realized they could capture these advantages in synthetic systems by encapsulating millimeter-scale cylindrical neodymium magnets inside molded silicone rubber shapes. |
In the resulting structures, the embedded magnets function as simplified bones, generating torque to bend the soft body when a magnetic field is applied. The magnetized cylinders can be oriented in customized patterns to achieve diverse modes of shape-change as the surrounding elastomer stretches and deforms. |
This approach effectively decouples actuation force from flexibility—the strong magnets efficiently transmit forces while the elastic matrix provides large strain capability and impact resilience. Crucially, the fabrication process is quite simple, requiring only low-cost commercial materials and standard molding procedures rather than specialized magnetization equipment. |
To showcase their bone-in-flesh actuators' versatility, the researchers created a variety of demonstration devices exhibiting useful behaviors. Auxetic structures that contract or expand in multiple directions were fabricated by embedding magnets into silicone membranes with arrays of holes and slots. |
Under a magnetic field, the rigid cylinders rotate to open or close the mesh-like geometries, producing dramatic changes in area and aspect ratio of up to 33% and 53% for contraction and expansion, respectively. These auxetic actuators completed their shape transformations within 0.2-0.4 seconds—comparable to the fastest previously reported magnetic soft actuators but with a greatly simplified manufacturing workflow. |
Other prototypes achieved dynamic 2D-to-3D shape transformations by tailoring the distributions of magnets and elastomer stiffness. In one example, a flat silicone disk with an optimized pattern of thick and thin regions deformed into a structure resembling a butterfly when actuated, increasing its height by 2.6 times while shrinking its diagonal projection by 28.5%. |
Another design utilized hinge-like folding of thin elastomer regions between thicker, magnet-embedded sections to create a structure evocative of the Bird's Nest Stadium in Beijing. |
A third prototype employed a two-layer elastomer sheet with embedded magnets arranged in specific orientations to produce a more complex origami-like folding pattern. Such structures could potentially serve as deployable devices or morphing surfaces. |
The team also exploited mechanical instabilities to construct actuators that could snap between distinct stable configurations under magnetic control. A bistable push-button mechanism switched between two stable states—one planar and one with a central pop-up dome. The transition between states occurred via rapid buckling of the elastomer, triggered by rotation of embedded magnets. |
Extending this concept, a multistable actuator comprising a cylindrical elastomer membrane with 24 embedded magnets demonstrated reversible switching among four stable configurations corresponding to progressively smaller diameters. These bistable and multistable actuators illustrate the potential for controlling discrete shape changes in soft devices. |
Finally, the researchers built a six-fingered gripper to demonstrate the potential for dexterous manipulation. Experiments showed the gripper could grasp and hold objects of various shapes and sizes, including a 3D printed letter weighing 0.3 grams, a peanut (0.8 grams), a screw bolt (4.5 grams), and a nut-and-bolt assembly weighing 9.0 grams. These tests illustrate the ability to grip and manipulate a range of small objects relevant to real-world applications. |
 |
a) Schematics of a six-fingered bone-in-flesh gripper, in top and 3D tilted views. b) FEA-predicted deformation in the tightening and the opening modes. Colors denote total displacement. c) Optical images demonstrating the grasping, transferring and release of a small object. d–f) Optical images of the gripper manipulating (d) a peanut, (e) a screw bolt and (f) a set of nut, washer and bolt. The magetic field strength is ?100 mT for (c–f). (Reprinted from DOI:10.1002/adfm.202401159, CC BY) |
The bone-in-flesh architecture offers several advantages over existing soft actuator technologies. The high magnetic energy density of the embedded neodymium cylinders, combined with their optimized integration into the flexible matrix, enables very fast actuation speeds comparable to the fastest prior magnetic soft actuators. At the same time, the compliant elastomer provides outstanding deformability and impact resistance, as confirmed by experiments and simulations showing the actuators can withstand severe mechanical insults like high-velocity collisions and compressions without damage. |
Crucially, these exceptional capabilities do not require complex fabrication: The parts can be manufactured via simple molding and magnet-insertion processes using only inexpensive commercial materials. |
By deploying insights from biological structures in a synthetic setting, the bone-in-flesh paradigm provides a template for creating high-performance soft machines that begin to close the gap with natural systems. The demonstrations in this work illustrate the ability to achieve useful functionalities like auxetic shape-change, planar-to-3D morphing, multi-stability, and dexterous manipulation along with rapid response times and robustness. Future developments could enable even more sophisticated behaviors by incorporating additional magnetic and structural elements to mimic the complexity of real organisms. |
Though still far from the intricacy and efficiency of living bodies, bone-in-flesh actuators point the way toward soft robotic systems that marry the speed and precision of conventional "hard" robots with the adaptability and resilience of biological creatures. |
Bridging this long-standing performance gap could ultimately produce soft machines that assist and interact with humans more safely and capably across medicine, industry, and beyond. At the same time, the work underscores the enduring value of biological inspiration as a wellspring of creative solutions to engineering challenges both old and new. |